Let me emphasize that citing these anthropic "coincidences" separately does not mean they are independent. One should not imagine, for example, that God picked out a value for the strength of hydrogen bonds and then, in a separate action, picked out atoms that could undergo hydrogen bonding. All the events covered by the term "anthropic coincidence" are jigsaw puzzle pieces in one big picture, a "TOE," Theory of Everything, crafted by God, the Grand Designer. Accordingly, it is a severe error to attempt to assign separate probabilities to individual coincidences and then multiply them together to get an overall possibility for the universe (but see Article V, "Is there a Probability for the Universe?"). Finally, the items listed below are by no means the only anthropic coincidences for chemistry; they are selected as critical representative examples.
Before discussing the chemistry required for life (as we know it), we should say a few words about what we mean by “life.”
What Is Life?
That's a question that philosophers and scientists have been arguing about for ages. It's also a question we should address before discussing what is required of chemistry, that life is enabled. So, here is a short list of essential properties of living things:
- Metabolism: living things take matter and energy from the environment to sustain themselves.
- Reproduction: living things beget, asexually or sexually, to yield descendants.
- Growth: living things change form during their existence.
The “Just Right” Properties of Chemistry
Before proceeding further, I want to acknowledge that this will be a condensed discussion. To complete a book or two on biochemistry, molecular biology, and physiology would be needed. In this article, I focus on hydrogen bonding, that weak bonding that is an essential property of water and biologically important molecules.
Here are two very general chemical properties required to enable living things to metabolize, reproduce, and grow:
- Complexity: to allow living things to carry out many different biological functions and transmit information within the organism and to descendants.
- "Just Right" Chemical Stability: If biologically essential molecules are too stable, metabolism and reproduction will be impossible; species will be inert, and necessary chemical reactions won't occur. Furthermore, if molecules are too reactive, then they will not exist long enough to carry out the required chemical functions.
In Part II, I mentioned some of the properties of water that support conditions for life. Here are some more.
The “Just Right” Solvent: Water
"The water that I shall give him will become in him a fountain of living water, welling up into eternal life. This is a new kind of water, a living, leaping water, welling up for those who are worthy. But why did Christ call the grace of the Spirit water? Because all things are dependent on water; plants and animals have their origin in water. Water comes down from heaven as rain, and although it is always the same in itself, it produces many different effects, one in the palm tree, another in the vine, and so on throughout the whole of creation. It does not come down, now as one thing, now as another, but while remaining essentially the same, it adapts itself to the needs of every creature that receives it."
—Quoted in the "Office of Readings" (Monday, Week 7 of Easter), from a catechetical instruction by St. Cyril of Jerusalem.
Water is called the "universal solvent" because it dissolves many kinds of molecules, enabling a variety of chemical reactions to occur. One property of water that aids solubility is hydrogen bonding discussed below. Another is the ability to form loosely bound complexes of water with the components of salts, positive and negative ions, as shown in the illustration below for dissolving salt, NaCl:
Sodium Chloride Dissolution by Andy Schmitz / CC BY 3.0 via Wikimedia Commons
In the diagram above, the slightly negatively charged oxygen part of H2O is attracted to the positively charged sodium ion, Na+; the slightly positively charged hydrogen part of H2O is attracted to the negatively charged chloride ion, Cl-. Loosely bound complexes of Na+ ion and water molecules and Cl- ion and water molecules are formed to be in solution. The illustration is diagrammatic (note: the sizes of ions and atoms are not to scale). The same type of solvation process applies to many other salts, compounds of potassium, calcium, and other metal ions that have roles in biochemistry.
“Just Right” Hydrogen Bond Strength: A Zipper, Not Glue
It has been recognized that hydrogen bonds restrain protein molecules to their native configurations, and I believe that as the methods of structural chemistry are further applied to physiological problems it will be found that the significance of the hydrogen bond for physiology is greater than that of any other single structural feature.
—Linus Pauling, "The Nature of the Chemical Bond"
Let's imagine God thinking about how He will design nature:
"Now I want chemistry to have not only strong interactions between atoms, but also gentle ones: so that complicated structures can unfold and rewind easily, and so that big and small molecules can come together and join for reactions and go apart readily—Velcro or a zipper, not glue or nails. What should I use? I have it—a hydrogen bond."
(Note: please don't criticize me for heresy here; I'm using a semi-literary device to make a point. I know God holds an infinite number of thoughts and plans simultaneously in His infinite mind.)
Here's the basic idea: H (hydrogen) bonded to O (oxygen) as in H-O-H (water) shows a slight positive electrical charge; :O, oxygen, with a pair of unbonded (lone) electrons (the two dots in front of the O), shows a slight negative charge. Similarly, N (nitrogen), with a pair of lone electrons, shows a slight negative charge, and N-H, hydrogen bonded to nitrogen, shows a slight positive charge. There is an electrical attraction between these small positive and negative charges; there is also, as NMR experiments have recently shown, a contribution from chemical bonding (sharing of electrons) to hydrogen bonding so that it is more than simple electrostatic interaction.
The figure below shows the types of hydrogen bonds important in molecules of biological interest.
The single dashes represent single bonds;
The “ = ” signs, represent double bonds;
The “ - - - ” represent hydrogen bonds;
The " : "represent lone pair electrons;
Superscripts, delta plus and delta minus, represent small net positive and negative charges.
Hydrogen bonds energies are about 1/20 to 1/30 the value of ordinary covalent bonds, so the hydrogen bonds can be broken much more easily than covalent bonds; for example, the O-H bond energy is about 430 kJoules/mole, whereas the O-H - - - :O hydrogen bond energy is 21 kJoules/mole.
I'll summarize the hydrogen bonds' role in biochemistry and molecular biology below.
“Just Right” Building Blocks for Proteins, Amino Acids
There are 20 amino acids that are the building blocks for proteins. Each amino acid molecule has a COOH, acidic carboxyl group (releases protons in solution), an NH2, basic amine group (accepts protons from solution) attached to a central carbon. Also attached to that group are 20 other different chemical parts (symbolized by "R"), as in the diagram below.
Peptide Formation Ball / Public domain, via Wikimedia Commons
The 20 amino acids that combine to build proteins are shown here. Proteins perform several essential functions:
- Catalysis, to speed up biochemical reactions (as enzymes).
- Transporting of smaller species through the organism and through cells (for example, hemoglobin transporting oxygen).
- Building material for tissue.
- Engines to move tissue and muscle.
- Antibodies to remove unwanted and dangerous stuff.
In all these functions, parts of the proteins bind loosely to other parts of the protein and thus form appropriate structures that are essential to their function. This is shown very nicely in this TED YouTube video, by Professor Ken Dill (SUNY/Stony Brook).
Another nice YouTube video showing protein flexibility is here. I should emphasize again that the flexibility and ability to form different structures are enabled by hydrogen bonds, bonds that can be zipped and unzipped readily, not glued or nailed. The diagram below illustrates how protein structures change when they act as a catalyst, that is, as an enzyme:
Allosteric competitive inhibition / CC BY-SA 3.0 via Wikimedia Commons
“Just Right” Hydrogen Bonding for Base Pairing in DNA
Watson (or was it Crick?), in a moment of insight, noticed that the bases (nitrogen-containing molecules bound to sugar pieces in nucleotides such as DNA and RNA) matched each other by hydrogen bonding like pieces in a jigsaw puzzle. They could thus stabilize a helical structure, by links across the spiral, as shown in the figure below.
There are four bases, adenine (A), cytosine (C), guanine (G), and thymine (T), organic compounds containing a nitrogen or amine group that can take on proteins. Hydrogen bonds are formed to link the members of a pair, as shown below.
Diagram Showing a Double Helix of a Chromosome by Cancer Research UK / CC BY-SA 4.0, via Wikimedia Commons
These bases are attached to sugar-type pieces, which in turn have phosphate groups on them that form the links between base units. The hydrogen bonds linking base pairs are strong enough to hold together the two DNA strands in the spiral helix, but weak enough that they can be "unzipped" by mild chemical action, an enzyme RNA polymerase, which yields messenger RNA.
Guanine (G) with Cytosine (C); Adenine (A) with Thymine (T); Iquo / CC BY-SA 3.0, via Wikimedia Commons
Alphabet Blocks to Code for Building Proteins
Before discussing the mechanism by which DNA enables protein synthesis, a few remarks are in order about the bases as letters in a word—words that encode which amino acids are used as building blocks in the protein. In this process, a linear combination of three bases is used to encode which amino acid is put into a protein. So we can regard the bases as letters and the combination of three bases as a three-letter word; the three-letter word is called a "codon". There are four bases, so there are 43 = 64 possible codons. There are twenty amino acids found in proteins, plus codons for beginning and ending protein synthesis, so that several codons may encode for incorporating the same amino acid, (i.e., there is a redundancy). See here and here for tables showing specific codon / amino acid relations.
Gene Expression—Transcription and Translation
I'll give just a brief summary here of gene expression—transcription and translation. More detailed accounts are given in the linked websites and videos.
Step 1: Transcription
RNA polymerase unzips the double strand and attaches complementary bases to single-strand RNA. See here and here. Note that RNA polymerase is a large protein, much bigger than the DNA strand. Also, note that one strand of the DNA serves as a "template"—bases complementary to bases in the template strand are linked (e.g., G to C, C to G, A to T, U to A), and as they are linked, they detach to yield mRNA (messenger RNA). See the flash animation for a more detailed description of this process.
Please see the linked animation—they will be well worth your while.
Step 2: Translation
mRNA leaves the cell nucleus and goes into the cytoplasm, where it attaches to a ribosome, where protein synthesis occurs. In the process of transfer, RNA molecules are sent by the ribosome to attach specific amino acids, coded by the mRNA, to form a protein.
Commentary
The description above is necessarily concise—a lot is left out, and I urge the reader to look at the recommended links, animations, and explanations and explore this fascinating subject. Summarizing gene expression in one paragraph is much like trying to do that for the Bible, Old and New Testaments.
What amazes me is that molecular biologists and those who deal with gene expression and all the other wonders of molecular biology do not think to paraphrase Psalm 19A: "[DNA] declares the glory of God, and [gene expression] shows forth the work of His hands. . .”
Certainly, the hydrogen bond, which is a crucial element in these processes, neither too weak nor too strong, is a marvel in itself.
God's providence in molecular biology is as marvelous as it is in physics.
What’s to Come
In Part V, I’ll examine what one can say (if anything) about the probability of all these anthropic coincidences.
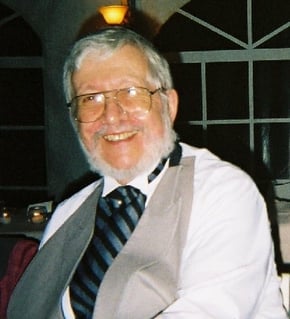